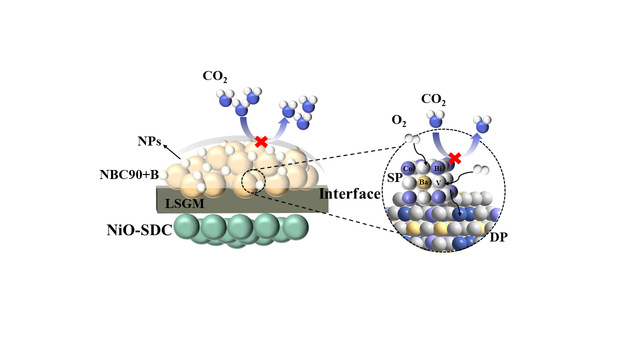
Figure 1 Working principle of heterostructures as cathodes of solid oxide fuel cells
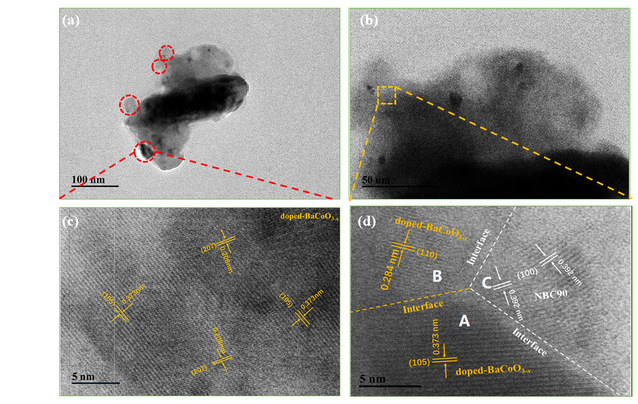
Figure 2 TEM analysis of NBC90+B: (a-b) bright field (BF)
TEM images; (c-d) HRTEM
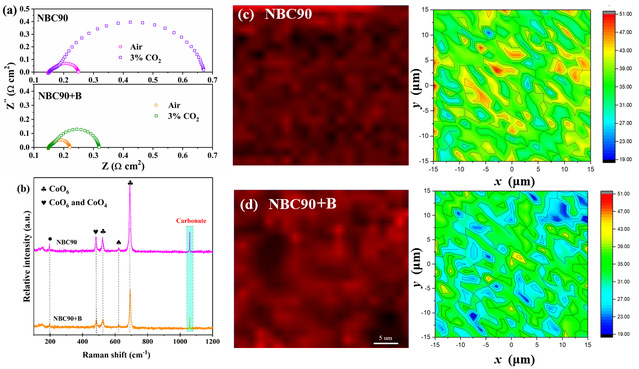
Figure 3 (a) Impedance contrast and (b) Raman spectra of NBC90 and NBC90+B
(treated under 3% CO2 for 12 hours); (c) Raman images of carbonate (~1,066 cm-1)
of NBC90 and (d) NBC90+B composite electrodes (after 12 hours under 3% CO2)
Perovskite-type oxides are characterized by their unique microstructure and exceptional electrochemical stability. These materials facilitate rapid oxygen transport kinetics and exhibit superior electrical conductivity, making them ideal candidates for cathode materials in solid oxide fuel cells. They are particularly adept at sustaining favorable electrochemical properties at moderate or even lower operating temperatures. The research team from the Institute of Coal-Based Fuel Cells and Efficient Hydrogen Production within the School of Materials Science and Physics has focused on the layered ordered LnBaCo2O5+δ (Ln=Nd) type double perovskite as their primary area of study. Through the creation of composite cathodes and the design of innovative material systems, they are exploring the interplay between electrode microstructure, electrochemical performance, and stability. Their goal is to develop novel layered double perovskite cathode materials.
The synthesis of the Nd0.9BaCo2O5+δ double perovskite oxide, featuring cation segregation, was achieved by controlling the defects at the Nd site. Concurrently, a Bi-ion trapping segregation oxide was also synthesized, resulting in a heterogeneous structure where the stable nano BaCo1-yBiyO3-x perovskite was anchored onto the Nd0.9BaCo2O5+δ double perovskite oxide. The study delved into the self-assembly process of this heterogeneous structure, examining the role of constructing heterogeneous interfaces in boosting the catalytic activity of the oxygen reduction reaction (ORR), enhancing stability, and improving CO2 tolerance. The research findings, titled “A new highly active and CO2-stable heterostructure cathode material for solid oxide fuel cells developed from bismuth ion-modified cation-deficient Nd0.9BaCo2O5+δ,” were published in the prestigious journal Applied Catalysis B: Environmental and Energy (with an impact factor of 20.2). Associate Professor Jin Fangjun from the School of Materials Science and Physics, CUMT is the lead author, with Professor Ling Yihan and Associate Professor Jin Fangjun serving as co-corresponding authors. CUMT is recognized as the primary institution for this work.
This study employs transmission electron microscopy (TEM) to further elucidate the surface microstructure of Nd0.9BaCo2O5+δ + BaCo1-yBiyO3-x (NBC90+B), as illustrated in Figure 2. The Fast Fourier Transform (FFT) images captured at various locations (A, B, C, and D) reveal potential lattice connections between the two phases: i) NPs (110) aligns with NBC90 (110); ii) There is a close lattice match between NPs (105) and NBC90 (100). A distinct heterogeneous interface between A|C and B|C can be discerned, which is instrumental in modulating the band gap structure and reducing the energy barrier for interface charge transfer. Furthermore, the lattice mismatch (~4.84%; between NPs (105) and NBC90 (100)) introduces oxygen dislocations and distortions at the interface, fostering a specific concentration of oxygen vacancies and facilitating enhanced ion diffusion. BaCo1-yBiyO3-x nanoparticles exhibit a preferential epitaxial growth along the NBC90 plane, maximizing their exposure to air. This anisotropic distribution also aids in lowering the oxygen dynamic potential energy, thereby accelerating the transport of oxygen ions. The electrochemical performance of the composite material is enhanced due to the increased concentration of oxygen vacancies within the lattice, a result of Nd3+ defects. Additionally, the surface activation effect of BaCo1-yBiyO3-x nanoparticles, which boosts surface oxygen vacancies, and the rapid oxygen ion transport capability of the heterostructure contribute to this improvement. Furthermore, the impedance test and Raman spectroscopy analysis conducted on NBC90+B in a CO2 environment revealed that the incorporation of highly acidic Bi ions into the lattice hindered the reaction and adsorption of acidic CO2, thereby reducing the formation of carbonates and improving resistance to CO2 poisoning. The long-term stability test showed that the single cell of NBC90+B could function effectively even after the introduction of 8% CO2, exhibiting a decay rate of only 0.54% per hour. Thanks to the unique assembly method of Bi ion modification and the synergistic effect of the constructed heterostructure, NBC90+B displayed outstanding oxygen reduction reaction (ORR) catalytic activity and CO2 stability.
The research team from the Institute of Coal-Based Fuel Cells and Efficient Hydrogen Production at the School of Materials Science and Physics has long been committed to the research of electrode materials, single cells, and systems aimed at efficient power generation and electrolytic water hydrogen production, contributing significantly to the realization of the carbon goals.